The reason I've held off this long is that this month marks the 50 year anniversary of one of the most important papers ever published: "Synthesis of Elements in Stars", by Burbidge, Burbidge, Fowler, and Hoyle (frequently abbreviated BBFH or B2FH).
The Big Bang model is only able to explain the existence of very light elements, such as hydrogen, helium, and trace amounts of lithium, and beryllium. Even if the model didn't predict this, it was apparent that older clusters of stars had less of these heavy elements present in their spectra. As such, it became clear that what astronomy needed was some mechanism to generate heavier elements from lighter ones. This is known as nucleosynthesis.
Thus, the paper asks,
What has been the history of the matter, on which we can make observations, which produce the elements and isotopes of that matter in the abundance distribution which observation yields?In other words, the goal of the paper was to explain how nature could possibly generate this distribution of elements:
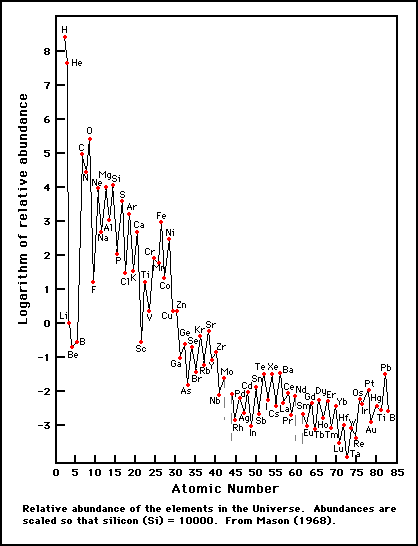
The story we tell in most introductory classes is horribly oversimplified, in some ways, to the point of being outright wrong. What's usually explained is that fusion builds up heavier and heavier elements in the cores of stars. When the star dies, either in a catastrophic supernova or in the slow death into a planetary nebula, those cooked up elements are released into the universe at large.
But there's a few problems with this. One of the most obvious is that either way, the real heavy elements are cooked up in the cores. Unless there's convection, they pretty much stay put. Whether the star explodes or quietly gives off it's out layers, those heavy elements don't do a lot of moving unless convection has brought them to the outer layers of the star.
Even if we assume that some of those heavy elements made it to the outer layers of the stars to be exploded off, or just gently released, there's still another major problem with the case in the former: Supernovae release a lot of energy. Enough energy that they can break up all those heavier nuclei that they just spent all that work making. This is known as photodisintegration.
So as it stands at that point, the only mechanism we have to really get more heavy elements is to build it up in stars, get it to the surface via convection, and then slowly release it after the red giant phase. But since the massive stars (the ones that can build elements much past carbon) explode, this can't account for the chemical abundances we observe! And even if astronomers dishonestly ignored all these little problems, there's still one more: Even in the cores of massive stars, you still don't cook up elements past iron. So where do all the elements heavier than those come from?
What the B2FH paper did, was to emphasize some alternative forms of heavy element generation. And it did so at great length. The B2FH paper is a book in its own right, taking over 100 pages to establish the novel processes that were being put together. (So the Creationist canard that they go to books to get their ideas across because laying the foundations for a good theory takes up too much space is nonsense. If you actually have a good theory, you can get space in journals.)
The two big ones are known as the s process and the r process, which stand for the slow process and the rapid process respectively. As the names suggest, one of the main differences is the time scales on which they occur.
The idea behind both processes is that in the right conditions, you can occasionally have a neutron smash its way into the nucleus of an atom. Sometimes that's not a big deal; It just creates a different isotope of the same element. However, some isotopes are more stable than others. The life of particular isotopes is frequently shown in the wonderfully scary looking chart of the nuclides.
Since I'm not sure how many people are familiar with this chart, I'd better do some explaining. Each column in this table is a particular element. Elements are uniquely defined by the number of protons the specific nucleus contains. The number of neutrons can change without changing the type of element it is. Rather, it just changes the isotope. But as I mentioned before, some isotopes are more stable than others.
In that particular chart, the gray isotopes running pretty much along the center are the stable forms. The further you get from this stable center, the less stable the atom is. If you're not really familiar with all this, you should probably be wondering what I mean by "stable". You can probably figure out that if something is stable, nothing really changes, but if it's unstable, what happens?
The answer is that one of the extra neutrons will undergo what's known as a "beta minus decay" (β−). This just means it gives off an electron. But if you're paying attention to your conservation of charge, you'll notice that we had a neutral particle, that just gave off a negatively charged particle, so we're going to need a positive one here somewhere. The answer is that the neutron became a proton (we see the reverse of this when we form neutron stars).
Since we now have another proton, that means instead of just having a different isotope, we now have an entirely different element; one that's one atomic number higher than the previous one.
Depending on how many neutrons are forcing their way in determines which process you're looking at. If the neutron flux is low (say one proton being captured per nuclei per ~10,000 years), then the chemical evolution is driven by the s-process. If it's high, then it's the r process.
Let's look at an example of how this works for the s process first:

First, we'll start with 109Ag (silver). Smack it with an extra neutron and it changes to 110Ag. But, you'll notice that the 110Ag doesn't last very long. According to the color key, it will decay in sometime less than a few years. Unless it's in some pretty special circumstance, it's not going to pick up another neutron before it decays. So it undergoes a beta decay and gets bumped up to 110Cd. Most of the isotopes up from there are pretty stable until it hits 115Cd. Then, again, it undergoes a beta decay and becomes 115In. The process continues on, building heavier elements as it goes.
So the next question is, where can things like this happen? Where are protons energetic enough and prevalent enough to force their way into a nucleus?
It turns out that during the red giant phase of a star's life, the conditions are just right in the outer layers of stars for this process to occur. Additionally, nebulae can be bombarded with neutrons as well, allowing this process to occur there as well. Slowly but surely, heavy elements are built up.
But there's some elements that the s process can't account for. For the sake of clarity, let's look at another part of that nuclide chart.

On this, you'll notice that in the column for Pd, there's a particular isotope (109Pd) that doesn't last very long. If we got there by the s process, it would decay to that 109Ag we started with earlier before being able to be transformed into the stable 110Pd. Thus, if there's any 110Pd in nature, the s process certainly can't account for it.
This is where the r process comes in. For the s process, the flux of neutrons is relatively low (<1011 neutrons per cm2 per second). Meanwhile, for the r process, we're talking about instances when the flux is more like 1022 neutrons per cm2 per second!
Thus, even for isotopes that don't last for more than a fraction of a second, the rate at which they're being bombarded is so high, that they can get stuck full of neutrons before they can decay. Thus, the element runs all the way down the column until it's so full of neutrons that it physically can't hold any more and any additional ones "drip" off.
Since it's impossible to keep up that high of a flux of neutrons forever, the rate will eventually die off and the decay process can begin. It takes elements diagonally up and to the right (the way this diagram is drawn) until it finds a stable element. The diagram for that looks something like this:

Thus, the r process can build up the heavier isotopes that the s process can't account for. The s process can build up the abundances near the inner part of the nuclide chart whereas the r process takes care of the outer, heavier isotopes.
So where in the universe do we find ridiculously high neutron fluxes? In supernovae! While the initial energy release can destroy heavy elements, the neutron flux remains sufficiently high for a good while longer and can build them right back up to even higher atomic numbers than the core was able to fuse in the first place.
But do these models hold up to observation? Sure they do. Both processes predict specific relative abundances. The distributions predicted match the observed abundances extremely well.
The B2FH paper laid the foundation for stellar nucleosynthesis 50 years ago at this point and has been well established for a long time now. But of course, dishonest creationists like Kent Hovind love to show that they're more closely related to ostriches than apes when they bury their heads in the sand and ask questions like this:
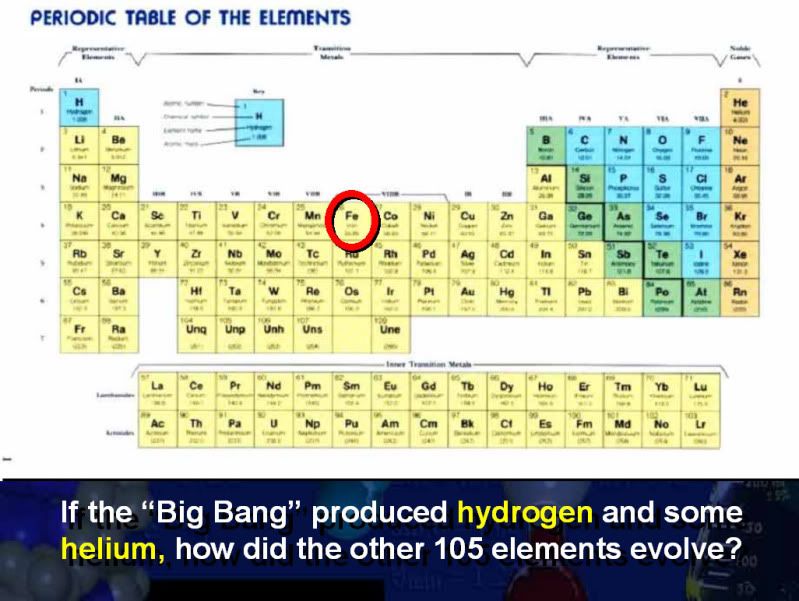
Thank you for such an understandable explanation.
ReplyDeleteSo at least one breakthrough was not a result of the space race. We've just had the Sputnik 50th anniversary - arguably the start of the space era.
ReplyDeleteI considered saying something about Sputnik's anniversary, but since the BA already took care of it (as well as all sorts of other sites) I figured I'd lay off the redundancy.
ReplyDeleteJust happened upon your blog when doing research for chemistry, and found it very helpful. Not only extremely well written and clear to understand, but explains complex ideas and processes without so much as a whiff of confusion! (especially for someone still on A-level Sciences) thanks alot :)
ReplyDeleteWow. I found this blog today (thanks to Mr. Plait :) and have learned a lot already! I had *no* idea about this, I thought supernova was the only explanation necassary. Can't wait to read the intro to astronomy series, thanks!
ReplyDeleteBestest post in this blog.. Brilliant and engrossing!
ReplyDeleteBestest post in this blog.. Brilliant and engrossing!
ReplyDeleteGreat Post!
ReplyDeleteThank you for such an understandable explanation.
ReplyDelete